The Burns lab works at the intersection of radiochemistry, inorganic chemistry, materials science and nanotechnology. We are interested in taking advantage of the nuclear property of radioactive decay to help address problems spanning from radiopharmaceuticals to nuclear power. In particular, our focus is on 1) the development of new technologies for used nuclear fuel recycling, 2) the investigation into emerging radioisotopes for medical applications, and 3) the design of nanomaterials for radiopharmaceuticals.
Hexavalent Co-Crystallization: A Single-Step Approach for Used Nuclear Fuel Recycle
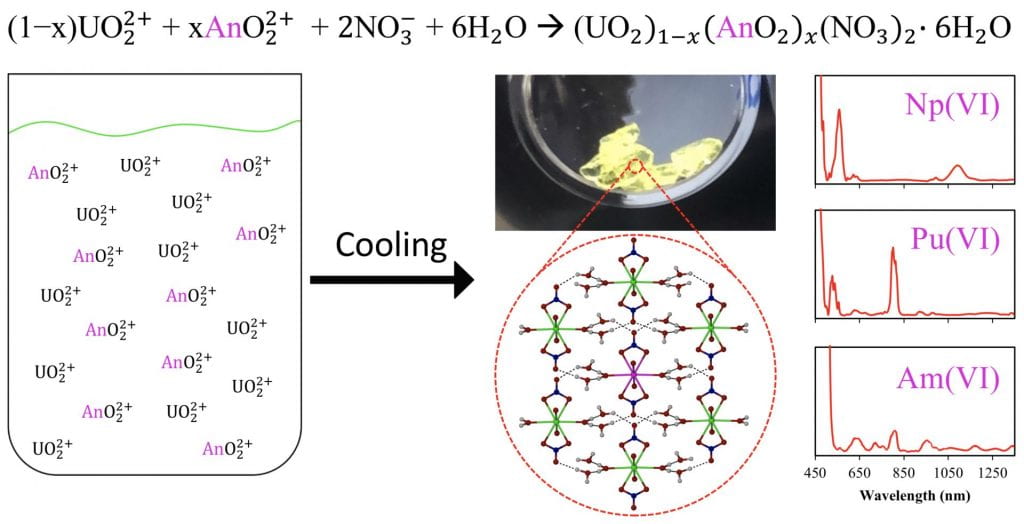
In this research we are focused on developing a new approach for a group actinide separation of used nuclear fuel (UNF)recycle based on the co-crystallization of oxidized transuranic (TRU) elements, Np, Pu, and Am, with uranyl nitrate hexahydrate (UNH), UO2(NO3)2•6H2O. This involves investigating the crystallization parameters of UNH in the presence of other metal species including common fission products like, Cs, Sr, Zr, and the lanthanides, as well as the TRUs, to understand the thermodynamics and kinetics of the process. Once isolated, the co-crystallized product provides a unique platform for the encapsulated oxidized An(V/VI) to be studied in the solid state. The redox chemistry of the TRUs, specifically Am, is a central focus, as only the oxidized actinides (An) will partition into the crystalline phase.
One of the greatest challenges facing the nuclear power industry is achieving a sustainable nuclear fuel cycle by implementing the recycling of UNF. It is critical that the processes used to recycle UNF maximize energy utilization of the fuel, minimize waste destined for geological storage, and address proliferation concerns. A single-step technology for recycle of UNF, which can recover the bulk of U and other TRU species as the first step after dissolution in nitric acid media, would be advantageous. We have been developing the hexavalent actinide co-crystallization, which is a single-step approach towards a Group An Extraction (GANEX) process. This single-step, group An separation would address issues surrounding process complexity of the current UNF recycling approaches, mainly the use of solvent extraction, by reducing the process to a single straightforward technology. The hexavalent actinide co-crystallization approach relies on the use of a single, mature separation method, crystallization, which selectively separates the hexavalent Ans U(VI), Np(VI), Pu(VI), and Am(VI) as a group out of solution containing dissolved UNF.
By a simple adjustment of temperature, the solubility of UO22+ can be significantly altered, resulting in a UNH precipitate. Other An(VI) species have been shown to behave in a similar fashion, and a scheme to co‑crystallize Np(VI), Pu(VI), and Am(VI) should be practical, taking advantage of the highly oxidizing environment that can be generated in strong nitric acid media. This new approach involving crystallization not only supports a GANEX-type approach, but it also provides a technique that does not involve organic compounds and influences the separation with only a thermal swing. Ultimately, this inherently proliferation-resistant approach has the potential to considerably reduce the number of steps in the recycle process of UNF, while significantly reducing or even eliminating the amount of secondary waste produced.
At-211 Development for Targeted Alpha Therapy

In this research, we pursue the discovery of the fundamental chemistry of astatine, including speciation, complex formation, and bonding of this interesting element. This knowledge is then applied to develop separation schemes and purification systems which can rapidly and efficiently separate, purify, and recover At for use.
Astatine is the rarest element on Earth. It occurs naturally only due to the radioactive decay of other elements in the earth’s crust. There are no known stable isotopes of astatine, and none of its isotopes has a half-life greater than 8 hours. This means the experimental design for astatine must be simple and rapid. One isotope of the element, At-211, has shown promise in cancer treatment in the area of targeted alpha therapy (TAT). TAT is a process in which an α-emitting radionuclide that is capable of high damage at a short range is attached to a molecule targeted at cancer cells. At-211 is of particular interest for TAT because it emits one α-particle and has a moderately-short half-life of 7.2 hours Although it is not widely available, At-211 has been used in a number of clinical trials.
The biggest challenge of recovering purified At-211 is the amount of time it takes and the need for sophisticated equipment that runs continuously. To overcome this challenge, we have recently developed a purification system based on a newly discovered chemical interaction of At with ketone molecules. This chemistry has been converted into a chromatography column to generate an elegant, continuous flow system and has resulted in a high purity, high yield recovery of At-211. The extraction chromatography system used in this project utilizes 3-octanone impregnated on porous beads. The results indicate that this process produces high-quality At-211 in about 10 to 20 minutes using minimal resources and mild chemicals, which should be compatible with human use. Using this new method of purification results in high yields of At-211 quicker than previous methods.
alpha-Zirconium Phosphate for use as Radiopharmaceuticals
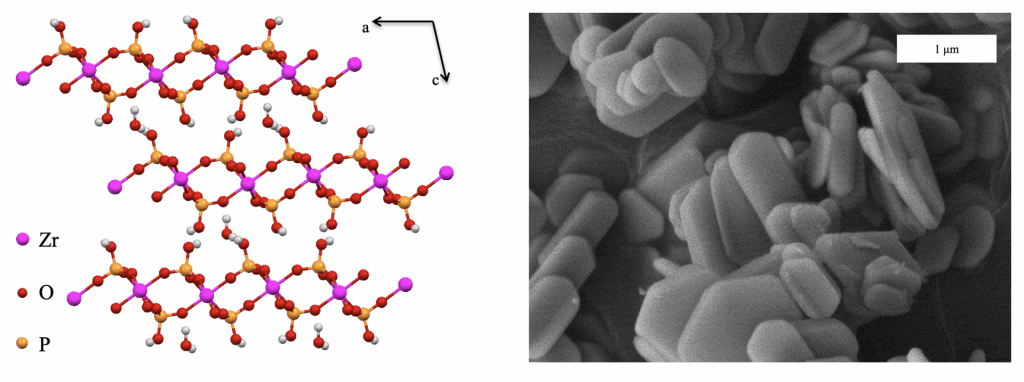
In this research, we are focused on investigating alpha-zirconium phosphate (α-ZrP) as a drug delivery mechanism for radionuclides. This includes gaining a fundamental understanding of aspects such as nanoparticle formation of the α-ZrP materials to achieve tunability in particle size and shape; the parameters which dictate the ion exchange properties to achieve, sufficient ion binding, high exchange capacity, and rapid kinetics; and the surface chemistry to label the particles and provide a vector to target specific cells or metabolic pathways within the body.
α-ZrP is an acidic layered cation exchange material with a composition of Zr(HPO4)2∙H2O. It can be described as a layered structure comprised of octahedrally coordinated zirconium atoms bound to six oxygen atoms from six different phosphate groups. The phosphate groups are bound to the layer by three oxygen atoms, with a protonated oxygen pointing towards the interlayer space. There is one-unit formula water molecule that hydrogen bonds the layers together through the protonated oxygen pointing towards the layers. The interlayer distance of α-ZrP is 7.6 Å. The proton on the oxygen dangling towards the interlayer space is acidic and therefore can participate in ion exchange reactions. The surface of α-ZrP can be modified through the functionalization of the surface phosphate groups by means of reaction with silanes, epoxides, isocyanates, and PEG chains.
α-ZrP can be produced as nanoplatelets, where the particle size and aspect ratio of these nanoplatelets can be tuned synthetically. This ability to synthesize these unique nanoplatelets, which have the capacity to have cations intercalated in between the layers, while possessing a diverse surface chemistry makes α-ZrP a prime candidate for study as a drug delivery vehicle. We are particularly interested in examining α-ZrP as a host for radionuclides which can be used as diagnostic and therapeutic agents.